Balancing building science and roof design
Thermal performance
Thermal bridges have an impact on the thermal performance of roofs and buildings. Fasteners can play a significant role in reducing the effective R-value of the building enclosure’s thermal layer.
Figure 5 shows roof fasteners acting as thermal bridges, melting the snow on the roof due to conductive heat transfer. Interestingly, the energy codes do not recognize the impact of thermal bridging from fasteners on continuous insulation systems. For this reason, designers should consider adhered roofing systems can be cost competitive once long-term energy efficiency is factored in.
It is also important to be cognizant of the negative effects of moisture-laden air, water vapour, and thermal bridges on long-term building performance, and to incorporate preventative measures into designs to mitigate risk. This requires a strong understanding of the building science principles.
Are minimum codes enough?
Architects can, and often should, design beyond the minimum code requirements within the National Building Code of Canada (NBC) and the National Energy Code of Canada for Buildings (NECB) to improve the durability and longevity of their buildings. This is especially important with respect to changing climate and increased frequency and magnitude of storms and weather events.
Designing building enclosures from a building science perspective can be an effective way to reduce condensation potential and moisture damage; reduce wind-related roof failures, for example, caused by corroded fasteners; and provide an energy-efficient thermal layer.
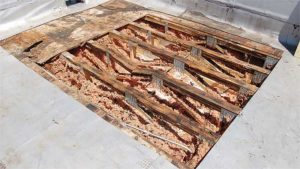
Designing with building science in mind
Today, architects are increasingly leveraging new technologies to design buildings of greater complexity. New materials are being incorporated, and traditional materials may be used in new ways. For example, the use of air barriers in roofs and walls is now mandated, so understanding how they can affect condensation potential is critical. The introduction of thermal insulation, tighter building enclosures, and increased mechanization of interior environments means the industry must change the way designs are approached and consider buildings as holistic systems rather than as individual parts.
When building science fundamentals are incorporated into designs, it not only increases the potential of long-term performance, improved durability, and energy efficiency, but also allows design ideas to align with an architect’s esthetic vision.
When buildings are not designed or constructed in accordance with sound building science, they are susceptible to:
∞ structural failures;
∞ building enclosure material degradation (Figure 5);
∞ biological growth; and
∞ reduced building life expectancy.
Practical ways to incorporate building science into designs
Designing a building in accordance with building science fundamentals is just the first step. Ensuring the as-constructed building aligns with the architect’s design intent is also important. The following are some steps the architect can take to meet these goals.
Design phase:
∞ Understand the impact of heat and moisture transfer through the building enclosure—hydrothermal analysis may be useful with more complex designs;
∞ Calculate temperature gradients across the building enclosure to determine the need and correct location of a vapour retarder/air barrier within the building enclosure; and
∞ Account for wind uplift and impact resistance, such as hail or wind-blown debris.